Hand Over Hand Steering A Professional Driver's Guide to Mastering The Perfect Racing Line
Hand Over Hand Steering A Professional Driver's Guide to Mastering The Perfect Racing Line - Racing Line Math The Critical 60 120 180 Degree Turn Calculations
When delving into the mathematics of a racing line, the focus shifts to understanding how specific turn angles—like 60, 120, or 180-degree corners—affect car behavior. The relationship between the sharpness of a corner, expressed through its radius, and the appropriate entry speed becomes crucial knowledge. Navigating these turns optimally requires a deep understanding of how to place the vehicle for maximum speed. The apex of a turn, that innermost point, acts as the focal point for achieving the best path through a corner. It's where optimal vehicle placement and acceleration are intertwined. Moreover, drivers learn to tailor their approach using different apex techniques—early or late apexing—to maximize cornering grip and control. By mastering these calculations, drivers gain a sharper appreciation for precision and adaptability, essential traits for pushing the limits of any vehicle's performance on the track. It's a continuous process that fine-tunes driver instincts and skills for handling various corner types within a complex circuit.
The optimal racing line through a corner isn't just about intuition; it involves a nuanced understanding of geometry and physics. A 60-degree turn, for instance, will require a different approach in terms of time and speed compared to a 180-degree corner, which often allows for smoother weight transfer and, consequently, better grip during acceleration.
The extreme forces experienced in high-performance vehicles during these maneuvers, particularly in the latest generation of supercars, are a crucial factor. Lateral G-forces can easily exceed 2G in critical turns, presenting a fascinating yet complex challenge for both driver and vehicle engineering. The calculations underpinning these forces are essential for designing and developing cars that can withstand such stress while maintaining driver safety.
Hitting the apex of a turn at the right moment is paramount. A small error, either being too early or too late, can result in a measurable difference in lap times. Telemetry data is increasingly used to understand these nuances, providing invaluable insight for tuning the vehicle and optimizing cornering. It's not uncommon to see lap time differences of a second or more over a mile-long circuit when drivers aren't hitting the apex precisely.
The importance of balancing a car's weight distribution can't be understated. Ideally, a 50/50 front-to-rear distribution, coupled with a lower center of gravity, provides more control and stability during rapid transitions through tight corners. This is especially important in scenarios where the car's balance could be challenged, like rapid transitions or unexpected surface changes.
Tire selection adds another layer of complexity. Different tire compounds, like softer compounds that might offer exceptional grip in a 120-degree turn, can overheat if not managed properly. This area touches upon a fascinating interplay of material science and thermodynamics, highlighting the need for careful tuning based on the track and conditions.
Suspension systems have a critical role to play in optimizing cornering. Variables like suspension travel and camber angles significantly affect how the tires interact with the road surface, influencing traction and ultimate performance. There’s a constant push and pull in design: how do you create suspension to handle the extreme demands of a supercar on a track but still maintain comfort on the street.
Performance vehicles often utilize variable steering ratios, allowing for greater finesse at lower speeds during tighter turns while maintaining rapid responsiveness at higher speeds. This engineering marvel reflects a delicate balancing act between mechanical precision and the driver's desired feel.
The role of aerodynamics becomes more pronounced as vehicles navigate turns at higher speeds. Downforce, a critical factor, can become the defining element of how cars handle. A higher level of downforce can significantly impact tire slip and influence the calculation of optimal entry and exit speeds.
Utilizing sophisticated simulation and predictive modelling techniques, engineers can preemptively adjust vehicle parameters based on expected conditions and driver behavior. Predicting a car’s path through a sequence of complex corners is a growing area of research.
Lastly, data logging technology plays a vital role in the evolution of vehicle performance. By capturing data like steering input and G-forces during testing or races, engineers gain valuable insights into vehicle and driver behaviors. This data fuels the development of increasingly sophisticated algorithms that optimize performance and uncover trends that lead to more effective strategies. The challenge is to translate all this data into something that is practical for real drivers.
Hand Over Hand Steering A Professional Driver's Guide to Mastering The Perfect Racing Line - Hand Position Blueprint How Formula 1 Drivers Place Their Grip at Speed
Understanding how Formula 1 drivers grip the steering wheel at high speeds is crucial for anyone seeking to master the perfect racing line. Their grip techniques are far from arbitrary; they're a carefully choreographed dance of hand movements designed for optimal control. Techniques like the push-pull method, the shuffle, and even rotational steering are employed to adapt grip depending on the demands of the corner or track section. It's not just about holding on; it's about seamlessly transitioning hand positions to maintain optimal steering control and maximize vehicle response. The modern Formula 1 steering wheel itself plays a role in this, with its multitude of buttons and controls that give drivers an incredible level of real-time feedback and adjustment during the race.
This detailed hand positioning blueprint is not simply a quirky quirk of F1; it exemplifies the interconnectedness between driver and machine. By precisely adjusting their grip, drivers not only enhance their vehicle control but also refine their understanding of car dynamics. It emphasizes that mastering vehicle behavior through nuanced control input is an essential ingredient in maximizing speed and performance on the track, a principle that can be applied to a wide range of vehicles, from luxury cars and high-performance vehicles to the latest supercars. It highlights that the driver's technique and feel for the car are key to truly unlocking a vehicle's performance potential.
Formula 1 drivers employ a unique hand position strategy that's a far cry from the typical 10 and 2 o'clock grip most drivers are used to. They often prefer a near-vertical hand position, akin to holding the wheel at 9 and 3 o'clock. This seemingly odd approach allows for a greater range of steering input and provides a more responsive feel at very high speeds. It's a bit counterintuitive, as it seems like it would be easier to oversteer with a more vertical grip, but the drivers have learned to control it and the engineers have designed systems to help them.
The level of control needed for F1 racing demands extremely precise hand movements. It’s not about huge arm movements, but subtle wrist adjustments that provide remarkable precision in the car's path. It is fascinating to observe how these drivers achieve such dexterity, which can reduce driver fatigue when those forces build up over time. It’s also not just about how drivers grip the wheel, but how they are moving their whole body to contribute to the desired result.
The physics behind it are interesting. There’s an interplay of forces and tire contact patches where hand position matters. Think about it, at speeds over 200 mph, even the tiniest change in hand position can have an influence on how the car responds, particularly in managing high cornering forces. I think that’s one area where the human-machine interface has to work flawlessly. It's incredible to think how critical the driver is to the outcome in these cars.
Modern steering systems provide drivers with some fantastic feedback via force feedback technology. It gives them a “feel” for the road surface and the tires through the steering wheel. That’s especially important when the speeds are high and conditions change unexpectedly, like in the latest generation of supercars, allowing for incredibly quick reaction time to minute changes in grip. It's a testament to how sophisticated the engineering has gotten in this area. It's easy to forget that the interface between the driver and the vehicle is critical for performance.
Intriguingly, the way a driver holds the steering wheel has an impact on the car's tires. In tight corners, maintaining optimal grip placement can actually reduce the load on the tires, thereby increasing the durability. It’s as if the steering wheel can make the tires last longer and maybe even perform slightly better. This seems to be an area of continuing research as tire compounds and technologies change.
Ergonomics have played a huge role in the evolution of steering wheel designs. Today, steering wheels are often custom-shaped and feature specialized surfaces that enhance grip and provide the driver with a lot of information and the ability to fine tune a vehicle. It’s very interesting to watch this interaction evolve. These are all about enhancing the overall handling experience, providing a better "connection" with the car.
When it comes to high-speed cornering, an F1 driver’s brain is remarkably adapted. Specific areas of the brain related to motor control and hand-eye coordination are actively involved during the process. The reactions and decision-making capabilities drivers develop in F1 racing are impressive. I imagine it takes thousands of hours of practice to achieve that level of control. They learn to steer and react at speeds that are hard to comprehend.
Race simulators are gaining popularity as a training tool. They allow drivers to rehearse intricate steering techniques and hand positions in various scenarios. It's a fantastic way to push drivers to their limits without the inherent risks of live racing. I also wonder how they deal with the emotional side of racing in a virtual setting.
The interior temperature of race cars can fluctuate wildly. The grip materials on steering wheels are designed to maintain their performance under both extreme heat and cold, which adds another layer of complexity to their design. Material scientists are continually pushing the boundaries here, striving to create the ideal grip that delivers predictable performance.
The interplay between the steering wheel and other components of the car, such as the suspension and tires, is highly complex. The engineering teams constantly fine-tune all of these systems to ensure they complement each other and allow for optimal performance. The goal is to provide a smooth, coordinated driving experience where each element seamlessly complements the other for high performance. It is also interesting to think about how they handle a variety of track conditions.
Hand Over Hand Steering A Professional Driver's Guide to Mastering The Perfect Racing Line - Corner Entry Speed Finding The Sweet Spot Between Braking and Turn In
Achieving optimal corner entry speed is crucial for maximizing performance in any performance vehicle, especially those meticulously tuned for the track. The key lies in harmonizing braking and turn-in, a delicate dance that can dramatically influence lap times. Finding the right balance requires a deep understanding of how the car responds under various conditions and how those conditions affect the corner's characteristics. A misstep, like braking too hard or entering at the wrong speed, can compromise grip and significantly impede progress through the corner, something that is amplified when considering the latest, extremely fast and capable supercars.
The ideal entry involves a seamless transition from braking to acceleration, strategically timed around the corner's apex. Modern supercars and luxury sports vehicles often leverage advanced chassis control and steering technologies to improve driver feedback and responsiveness, yet even these systems benefit from a skilled hand. Ultimately, the precise interaction between braking and turn-in is a testament to both the driver's prowess and the engineering marvels that underpin these high-performance vehicles. There's always a constant tension between the driver and the technology, and that's what makes the process both exciting and challenging.
Entering a corner at the right speed is about finding the sweet spot between braking and turning the steering wheel. Research suggests that, rather than slamming on the brakes, it's more effective to gradually apply them, maximizing deceleration while preparing for the turn. This emphasizes the crucial role of timing and driver skill.
The physics of high-speed cornering generate considerable forces, with lateral G-forces regularly exceeding 3G in the world of professional racing. This highlights the remarkable engineering that goes into both the suspension and tire technology used in these vehicles, allowing them to handle these extreme forces and maintain performance.
As a car enters a turn, there's a significant shift in weight distribution, which can impact handling. A reduction in grip at the rear tires can lead to oversteer, demanding that drivers have a precise understanding of when to modulate braking and acceleration to maintain control.
Tire performance is greatly impacted by temperature. Racing tire compounds are developed to function optimally within a specific temperature window. Managing corner entry speed involves quickly getting tires up to temperature without causing them to overheat, which can degrade performance and tire consistency.
Suspension design is incredibly complex, involving extensive computational models to account for the dynamic changes in weight as the car navigates a turn while keeping tires firmly on the ground. Even small changes in suspension geometry can dramatically affect a car's behavior during corner entry.
At higher speeds, downforce generated by aerodynamic elements significantly affects a car's cornering ability. Interestingly, increasing downforce might actually lead to a slight reduction in the ideal entry speed because it increases the grip available during braking and turning.
The role of the throttle during corner entry is often overlooked, but research suggests it's as critical as braking. Carefully adjusting the throttle can affect weight distribution, leading to better control during the turn and influencing exit speed.
Modern racing vehicles are equipped with a myriad of sensors that provide real-time data on aspects like yaw, pitch, and roll. This data offers drivers and engineers valuable information that can inform corner entry strategies and adjustments to the car's suspension settings, adapting to specific track conditions.
The stiffness of a vehicle's chassis plays a critical role in cornering behavior. There's a growing understanding that excessive chassis rigidity can lead to a lack of feedback through the steering, while a carefully engineered level of flex can enhance feedback, improving driver confidence during corner entry.
Studies into how drivers respond to situations in corners reveal that the speed at which our brains process information impacts performance. Variations in reaction times between drivers highlight the importance of practice. Consistent practice cultivates more intuitive decision-making during those high-pressure moments, leading to optimized corner handling.
Hand Over Hand Steering A Professional Driver's Guide to Mastering The Perfect Racing Line - Apex Hunting A Technical Analysis of Mid Corner Racing Lines
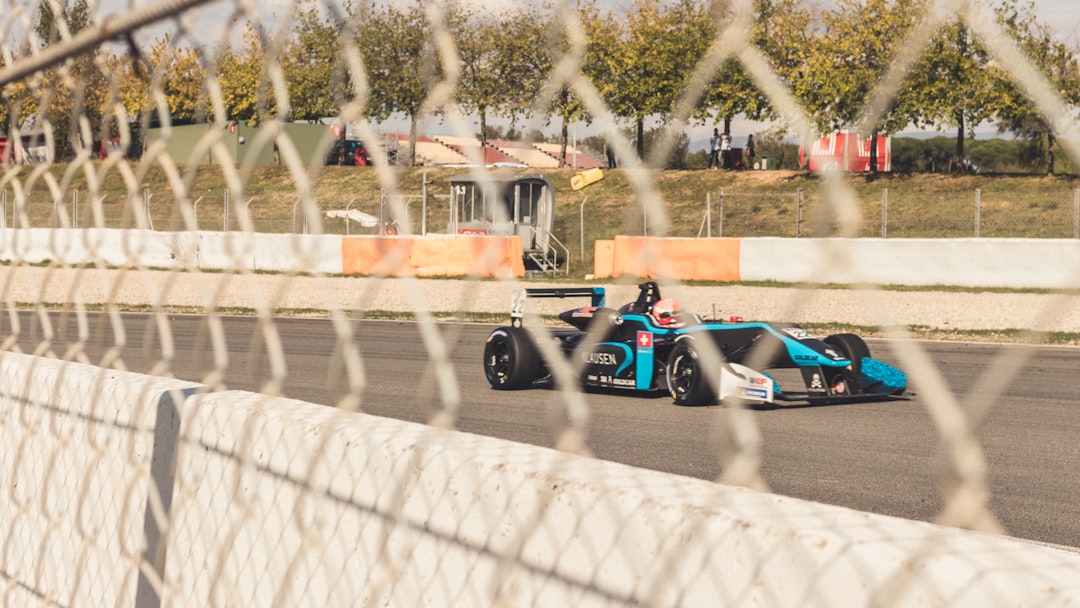
The core of "Apex Hunting: A Technical Analysis of Mid Corner Racing Lines" revolves around optimizing a vehicle's path through the middle section of a turn. The apex, the innermost point of a corner, becomes the focal point for maximizing speed and minimizing distance traveled. Finding the optimal racing line within a corner isn't simply about intuition; it requires a deep understanding of how the car behaves under various conditions. This understanding is particularly important with the latest supercar models, which offer incredible grip and acceleration but require a skilled touch to truly exploit their potential.
Drivers can adapt their approach depending on track characteristics, vehicle type, and desired speed. This often involves fine-tuning the point where a driver aims for the apex – whether choosing an earlier or later apex based on the curve's geometry and the vehicle's performance characteristics. This dynamic interplay between driver skill and the latest advancements in supercar engineering—things like suspension, aerodynamics, and advanced chassis systems—is at the heart of achieving peak performance on the track. The result? Smoother transitions, better acceleration out of the corner, and ultimately, improved lap times. It's a delicate dance of inputs that showcases the close relationship between the car's capabilities and the driver's ability to extract the most from them.
The pursuit of the perfect racing line in modern performance vehicles has led to fascinating engineering advancements. Consider the revolutionary suspension geometry found in cars like the Ferrari SF90 Stradale. These systems dynamically adjust suspension settings during cornering, maximizing tire contact and significantly improving grip and cornering stability. It's a testament to how much vehicle dynamics has changed in recent years.
Similarly, the role of aerodynamics at high speeds has become increasingly vital. Cars like the McLaren P1 can generate over 1,000 pounds of downforce at 150 mph, allowing them to corner with phenomenal precision. This raises questions about the optimal balance between downforce and drag, an area where advanced computational fluid dynamics is critical in the design process.
Another area of innovation lies in tire technology. High-performance tires are engineered with specific bulk modulus properties, meaning they can adapt to the heat and pressure changes that occur during cornering. This feature, especially vital in ultra-high-performance models, ensures they can maintain grip levels even when faced with rapid shifts in cornering forces.
Professional racing puts enormous stress on cars. The lateral G-forces experienced in these cars can exceed 5G during cornering. As a result, the construction of chassis and components has become more critical. Engineers now rely on advanced materials like carbon fiber and reinforced composites to withstand these extreme forces and protect the driver.
Predictive analytics is changing the way cars are developed. By employing machine learning algorithms, development teams can simulate various driving scenarios and predict optimal vehicle settings for any track. This ability to "tune" suspension and aerodynamic components before even going to the track reduces the amount of on-track testing required, potentially speeding up development.
Driver feedback technologies have reached another level with force feedback systems found in modern steering wheels. These systems are designed to mimic the road feel in real time, allowing drivers to detect even the slightest changes in grip levels at very high speed. This technology gives an edge to professional drivers by sharpening their reaction times and improving their handling skills.
How weight shifts during cornering has a big impact on how a car handles. The way a car is engineered to maintain optimal tire contact despite this weight transfer can be the difference in setting fast times. Recent innovations have focused on lowering the center of gravity, particularly in high-performance models, to enhance this effect.
Chassis design is also being rethought. There's a growing awareness that a certain degree of chassis flex can improve driver feedback and handling. This is counter to the old belief that a stiffer chassis was always better. In fact, a bit of calculated flex can improve the driver's sense of what the car is doing and make the experience more engaging.
Racing brake systems found in vehicles like the Aston Martin Valkyrie incorporate sophisticated thermal management. This technology helps prevent brake fade, even after multiple high-speed stops, ensuring reliable braking performance in challenging race conditions. It's an interesting example of how thermal dynamics can improve the reliability of a vehicle system.
Lastly, the incredible physical demands of professional racing have measurable effects on a driver’s physiology over time. They develop faster reflexes and their ability to process information increases. Some research suggests that motorsport simulator training can condition the nervous system to respond more quickly, providing a tangible advantage in high-stakes situations.
The pursuit of the perfect racing line continues to drive innovation in automotive engineering. From dynamic suspension to advanced driver feedback, these advancements reflect the complex interplay of physics, materials science, and driver skill. And as the sport and technology continue to evolve, it will be fascinating to see what innovations emerge in the future.
Hand Over Hand Steering A Professional Driver's Guide to Mastering The Perfect Racing Line - Track Exit Acceleration The Physics Behind Quick Corner Release
"Track Exit Acceleration: The Physics Behind Quick Corner Release" explores the critical final stage of cornering, where maximizing acceleration after the apex can significantly impact lap times. Modern advancements in steering technology and performance engineering have allowed drivers to fine-tune their exit speeds, transforming what might seem like simple acceleration into a nuanced skill linked to the vehicle's overall dynamics. It highlights that the gap between a successful and less-than-ideal corner exit often boils down to understanding the interplay of weight transfer, maintaining a balanced distribution of friction, and even managing tire temperatures. Gaining insights into specific vehicle traits emphasizes the need for drivers to tailor their techniques, making the most of the potential found in contemporary supercars and performance vehicles when negotiating challenging race circuits. This concluding stage of cornering not only demands accuracy and timing but also a thorough comprehension of the fundamental physics involved in high-speed driving.
Track exit acceleration is essentially a short drag race from the apex towards the finish line. Even small variations in speed can have a surprisingly large impact on lap times. If you drop from 110.3 mph to 109.3 mph, it can cost you up to 0.13 seconds on the lap. This emphasizes the importance of getting every little detail right. The physics of corner exit acceleration highlight just how sensitive a racing car's performance can be.
The physics of drag racing can be applied to help us understand how to maximize corner exit speed. In essence, the goal is to generate the highest possible acceleration as the car exits the turn. But getting that right isn't as simple as just slamming on the gas.
The design of a car's chassis becomes extremely important during these high-speed, high-force moments. Take modern supercars, like those using carbon fiber materials. These materials can handle some incredibly intense forces. The cars built today can experience lateral G-forces in the neighborhood of 5G in a corner exit. That's extreme and it puts a strain on both the car and the driver. The design of the car must be such that it can withstand the stress of these huge forces for a prolonged period. We don't want the chassis to begin to flex beyond its intended performance. This has driven the use of advanced materials in luxury car chassis design in the past couple of years.
One of the critical elements of achieving optimal corner exit acceleration is proper tire temperature management. For high-performance race tires, like those found on modern supercars, there is a relatively narrow window of ideal operating temperature, generally between 80°C to 100°C. Going outside this window results in degraded grip which reduces the effectiveness of the vehicle's acceleration during corner exit. This highlights the importance of understanding the nuances of tire temperature throughout the entire cornering process.
The latest advancements in aerodynamics have completely changed how supercars corner. Look at cars like the McLaren P1; it's able to generate over 1,000 pounds of downforce at 150 mph. Downforce can actually significantly impact acceleration and is a crucial factor in maintaining stability through the corner and then on the exit. The car is practically glued to the road. This also impacts how designers think about drag, a factor that always works against acceleration. The relationship between downforce and drag is an important area of engineering and design for modern supercars.
Suspension geometry is another area that's been significantly innovated. Cars like the Ferrari SF90 Stradale use sophisticated suspension systems that actively adjust throughout the cornering process. By optimizing tire contact patch throughout the corner and exit, it maximizes grip and stability, which in turn allows the car to accelerate harder on the corner exit. This is an example of how active suspension is changing the way vehicles are designed and performing on the track.
Brake system engineering is as vital for exiting a corner as it is for entering. Look at the Aston Martin Valkyrie's thermal management system. It's designed to prevent brake fade during intense racing conditions, meaning brakes can handle the heat that comes from prolonged high-speed driving and cornering. This keeps brake performance consistent and reliable, which is crucial for the driver to optimize acceleration exiting the corner. Brakes can fade in performance if they overheat, and that can cost time in the race.
We might think of a car as a stiff structure, but that's not always the case in performance car design. There is a growing awareness that a certain amount of controlled chassis flex can benefit the driver by providing them with a better 'feel' of what the car is doing. It makes it easier for the driver to make corrections to keep the car on track. This flies in the face of the traditional thinking about chassis design. The older view was that a stiffer chassis was always better. It seems we now realize that stiffening chassis components may not always improve driver feedback or driver performance.
Simulation techniques are changing the way race cars are developed. With machine learning and other forms of simulation, vehicle engineers can virtually explore millions of corner scenarios, vehicle setups, and driver behavior without ever needing to step on a racetrack. This type of pre-testing can quickly lead to optimal vehicle settings for any track. This also minimizes the need for extensive on-track testing and may ultimately lead to vehicles that are closer to their maximum performance from the day they are released. It's truly changed how things are done.
Force feedback technology has advanced substantially in the past few years. This gives the driver almost real-time information about what's happening between the tire and the track surface. That's especially useful during the corner exit when forces are intense. It can allow a driver to make adjustments with lightning-fast reaction times that would be impossible without this information. This technology will undoubtedly keep growing in the future.
The way a car's weight shifts during the corner and through the exit is extremely important. Engineers have found that lowering a vehicle's center of gravity can result in significant improvements in overall handling performance, especially during high-speed cornering. Lowering the center of gravity improves the ability to keep tires on the road during weight shifts, which, in turn, improves acceleration out of the corner.
Humans have the ability to adapt. It’s impressive what happens to the human body when it's subjected to the stresses of high-performance racing. It’s become evident that professional racers develop faster reflexes, better ability to process information, and they develop an intuitive ability to handle cornering situations. Research shows that drivers who use simulators in their training programs show improvement in reflexes and the speed with which they process sensory information. This suggests that simulator training may provide a tangible benefit to those drivers who use them.
Mastering corner exit acceleration is about blending all these things together, which is a complex feat that requires a combination of car engineering and driver talent. We can expect to see the continued advancement in materials science, software, and engineering that will only drive innovation in the field
Hand Over Hand Steering A Professional Driver's Guide to Mastering The Perfect Racing Line - Steering Wheel Management Advanced Hand Movement Timing During High G Forces
When dealing with the intense forces encountered in high-performance driving, particularly in modern supercars, steering wheel control becomes a crucial skill. The hand-over-hand steering method proves especially valuable during high-G cornering scenarios. It's a technique where drivers cross their hands over the wheel to smoothly shift grip and make quick, precise steering inputs. This is important because these vehicles can subject drivers to extreme lateral forces, making it vital to manage the steering wheel effectively. This quick, precise control is only possible by developing a highly responsive hand movement. The ability to swiftly and smoothly shift hand positions enables drivers to react quickly and effectively counter the physics at play during extreme cornering. The effectiveness of this hand-over-hand technique underscores the delicate dance between driver skill and the design of modern high-performance vehicles, where precision control is paramount for maintaining speed and achieving optimal performance on the track. It also showcases the growing sophistication of supercar design that is continually demanding more precise control input.
During high-G situations, which are common in performance driving, particularly with supercars, a driver's reaction time can suffer. Studies have suggested that the high forces, especially when lateral G-forces surpass 2G, can lead to a lag in perception, making split-second decisions trickier while cornering. This is something that engineers really need to keep in mind when designing the driver-vehicle interface.
Modern supercars like the Lamborghini Sián often feature dynamic steering systems that adapt the steering ratio depending on speed and how much the driver turns the wheel. This approach makes for more precise handling at low speeds, such as when parking or maneuvering in tight spaces, while also offering a quicker response at high speeds. It improves a driver's sense of control in high-G situations, but it raises questions about the overall design of the system.
The development of high-performance tires, like those found on the Lamborghini Sián, has led to remarkable progress in heat management. These tires can achieve their optimal operating temperature in a short period, perhaps as quickly as under a minute, allowing for maximum grip during high-performance driving. It minimizes the risk of the tires overheating during periods of intense cornering, which can impact performance. This is crucial since the tire's temperature is a factor in maximizing grip and stability.
Active suspension systems, like those in the Bugatti Chiron, are able to adjust continuously during cornering. They can instantaneously modify damping and spring rates to reduce body roll and maintain the tires' contact with the road surface. This is absolutely critical during high G-forces, as the tires' grip is essential for maintaining control of the vehicle. It’s impressive how quickly the system can adjust.
In the design of high-performance vehicles, driver ergonomics are incredibly important. A well-designed cockpit can help drivers avoid becoming fatigued, even under physically challenging conditions, such as when cornering at high G-forces. They do this with features like well-placed steering mechanisms and enhanced seat support. It's fascinating how much work goes into designing these aspects of a car.
The established thinking used to be that a stiff chassis meant the best performance. However, recent research indicates that introducing a controlled amount of chassis flex might actually provide the driver with a stronger sense of the car's behavior during extreme events, like when cornering with high G-forces. The driver gains a better feel for what the car is doing and, consequently, better control. It's another example of how engineering assumptions can change over time.
Engineers are now employing sophisticated AI-driven predictive models to simulate a vehicle's performance through various cornering situations. This allows them to fine-tune suspension setups, potentially maximizing grip and stability before the car even goes on the track. This ability to optimize suspension before physical testing could become extremely important for a racing team, as it can give them a significant competitive advantage.
Vehicles like the Rimac Nevera have shown that advanced materials, like composite materials, are transforming chassis design. These materials provide lightweight structures that can withstand enormous lateral forces. They improve acceleration, handling, and overall vehicle safety during high-G situations. It’s remarkable how these materials have changed the way vehicles are designed.
Modern steering wheel technology has advanced to the point that it provides a direct feedback loop between a car's dynamic responses and a driver's steering adjustments. Force feedback steering systems tell the driver how quickly the car reacts to steering adjustments. This is essential for maintaining control during high-speed turns, as drivers need to be able to quickly make changes to stay on track. It’s another example of the importance of driver feedback.
It’s been noted that professional racers develop remarkable adaptations over time in their nervous systems due to the physically demanding nature of motorsport. Through consistent exposure to intense forces and rapid decision-making, they improve both their reflexes and cognitive processing speed. This is especially important in racing environments where quick decisions under pressure are critical to success. It’s another example of how human physiology adapts to demanding conditions.
More Posts from tunedbyai.io: